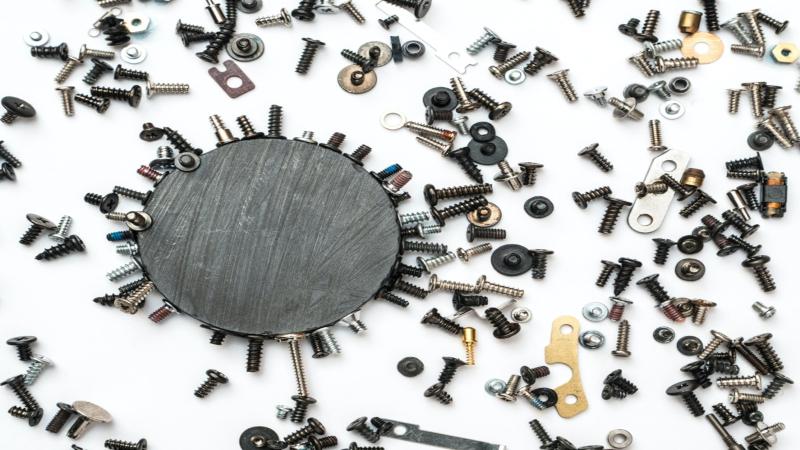
Digital signals are the backbone of communication systems. The signals, a combination of zeroes and ones, are processed by hardware systems. The hardware systems transmit and receive the signals with the help of intermediate systems, which scientists called ‘digital receiver systems’ or DRS. These digital receivers systems can perform various functions on the signals, like amplifying them, filtering them, or adding them together. Scientists choose the mathematical operations on the signals according to the particular applications they are interested in.
The hardware of digital receiver systems are built with standard silicon-based memory devices. Researchers implement computer codes that make these devices perform mathematical operations on the signal they receive. It enables digital receiver systems to measure fundamental properties of matter. ‘Spin’ is one such property, and the spin of electrons determines the magnetism of most of the objects that we have around us on Earth. When magnetic matter creates signals, analysing them with DRS lets scientists study the magnetic fields. Analysing the properties of the signals, for example, how they vary with time, scientists can measure the fields and study their small fluctuations.
In a new study, scientists from Raman Research Institute (RRI), Bengaluru, have devised a more efficient, faster, and low-cost digital receiver system that can make precise measurements of magnetic fields. The study was supported by the Ministry of Electronics and Information Technology (MeitY) and the Department of Science and Technology, Government of India. It was published in the journal IEEE Transactions on Instrumentation and Measurement. The system costs less than 350$ for all the silicon-based hardware and associated software.
“The electrons’ spin is not constant at room temperatures. They fluctuate,” explains Saptarishi Chaudhuri, associate professor of RRI and a co-author of the study.
These spin-fluctuations cause what scientists call ‘spin-noise’. By measuring the tiny fluctuations in the magnetic field, the researchers can infer the spin-noise accurately.
The work is an extension of the PhD thesis work of the co-authors Maheswar Swar and Subhajit Bhar of RRI. The researchers heated rubidium atoms to temperatures ranging between 100 and 200 degree Celsius, causing spin-fluctuations. Then, they bombarded the atoms with a laser, which has a property called ‘polarization’, a fundamental property of light. The spin-fluctuations caused the laser’s polarization to fluctuate, which the researchers measured using a light detector. The polarization fluctuation is the signal for the digital receiver system. They then designed the system to work in two different modes.
One of them uses a widely-used mathematical function, the ‘Fourier transform’ of the signal. The function is named after its inventor Joseph Fourier. The Fourier transform of the signal lets them calculate how the rubidium atom’s energies vary, from which they can directly infer the magnetic field. A standard method of measuring the magnetic field analyses small frequency ranges of the signal separately. The researchers showed that their method speeds up the calculations compared to the standard method. Their improved method also increased their confidence in how the electrons’ energies vary more than ten times.
Sometimes, while measuring magnetic fields, the DRS may receive signals only for a short time. In such scenarios, it is essential to record the signal as it gets created without losing any part of it. The researchers successfully implemented this ability with the help of a combination of standard hardware and computer codes. They measured a magnetic field of 800 microgauss –– roughly a thousand times smaller than the Earth’s magnetic field, within a tenth of a second.
There was, however, a problem –– electromagnetic interference to the signal the DRS receives. “The source is the power supply to the digital receiver, and radio-frequency signals emanating from other nearby electronic devices, such as the computers, phones, lasers and other laboratory instruments,” explains V. Mugundhan, another co-author of the study. They got rid of these sources by using a battery bank to power the DRS’s hardware components and shielded them entirely from interference using a 5-millimetre thick layer of mild steel. “We have also developed high-end data processing algorithms to remove the residual interference,” he adds.
The researchers applied an external magnetic field across the heated rubidium atoms. They demonstrated that their measurement of the magnetic field was consistent with what they expected. Thus, they demonstrated that their two-component digital receiver system works as an atomic magnetometer.
“Our magnetometer can be deployed to measure unknown magnetic fields,” says Saptarishi.
Having demonstrated the functioning of a digital receiver system to precisely measure atomic magnetic fields, the researchers are open to large-scale manufacturing or commercialisation of the device. Such a step would require partners in the industry to show interest in the project.
“There are no bottlenecks in manufacturing our device on large scales,” Saptarishi signs off.
This article has been run past the researchers, whose work is covered, to ensure accuracy.
This work has been previously published here.