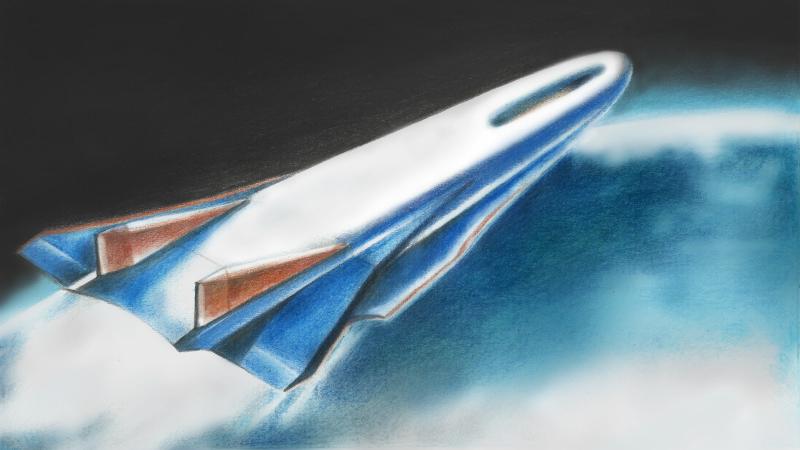
How basic academic research in the country can help in realizing the dream of designing a reusable hypersonic aircraft.
What if you could fly from Mumbai to New York in just 2.5 hours? If manned hypersonic flights were a reality, you wouldn’t have to spend about 18 long hours in a cramped aeroplane! Research works led by Prof. Shripad P. Mahulikar from the Aerospace Department, Indian Institute of Technology Bombay (IITB) are contributing to setting up a foundation to make hypersonic aeroplane design a reality. The researchers have proposed changes to the aircraft geometry to address the problem of heating of the body at the extremely high speed.
Hypersonic aeroplanes, meaning aeroplanes that fly several times faster than sound, are the ‘next-big-thing’ in space and military aerospace research across the globe. For example, the company Space-X has announced its plans for building hypersonic reusable launch vehicles and China has successfully tested hypersonic missiles. Russia and USA have full-fledged hypersonic research programs underway.
“Reusable Hypersonic Vehicles (RHVs) are promising low-cost candidates for future space missions”, remarks Prof. Mahulikar. “The future of space missions will be based on the cost reduction for a kilogram of payload (satellite) launched”, he says, adding that this can be achieved by the Single-Stage-to-Orbit (SSTO) RHVs. These vehicles are ‘fully-reusable’ as they reach the intended orbit without expending their hardware. “The future of military missions will also get a boost with hypersonic attack vehicles that can achieve their offensive mission with an unprecedented surprise element due to the high speed”, says Prof. Mahulikar.
The RHVs fly at enormous speeds (more than 6000 kmph) which is greater than five times (known as Mach 5) the speed of sound and at a typical cruise altitude exceeding 35-km! In comparison, currently operational long-distance commercial aeroplanes such as the Boeing-747 are subsonic, and cruise at about Mach 0.8 (less than 1000 kmph) at a cruise altitude of about 11-km.
There are unprecedented challenges in designing such RHVs due to the high speeds. To achieve hypersonic speeds, these planes need specialized engines, efficient management of the heating due to the air resistance, and changes to the configuration design of the aircraft. Prof. Mahulikar’s basic research findings on, “Aerothermal Considerations in Configuration Design of RHVs”, provides clues and insights into cracking some of these challenges.
Hurdles to flight at hypersonic speeds
The air-flow over an aircraft creates an aerodynamic drag force which resists the aircraft’s forward motion. Aircraft wings are designed to minimise the air-drag which reduces the fuel consumed. Aircraft designers modify the aircraft geometry to achieve this with insignificant repercussions to its weight.
The aerodynamic drag also heats the aircraft’s body and is known as aerodynamic heating. At hypersonic speeds, the aerodynamic heating can increase the vehicle temperatures to higher than 1600°C. The designer’s focus then shifts from designing just for aerodynamic drag reduction to designing for managing the harsh aerothermal environment. Studying and understanding the aerothermal environment for RHVs enables designing a reliable Thermal Protection System (TPS) – the lifeline of hypersonic aircraft.
Solving the hypersonic vehicle’s aerodynamic heating challenge
At speeds greater than sound a different drag comes into play (caused by the shock waves) and designers address this drag by incorporating a ‘sweepback’ to the wings, i.e. a wing that angles backwards from where it is joined to the aircraft’s body (fuselage). The angle at which the wing is ‘sweptback’ varies from 0° (no sweep) for straight-winged low-speed aircraft to about 45° and beyond for supersonic aircraft such as the fighter jets (e.g. F-16). The sweepback angle is designed to minimize aerodynamic drag and its value for minimum drag is the ‘drag-minimized sweepback’.
Figure 1: Concept of Sweepback Angle
Prof. Mahulikar proposed that due to aerothermal considerations, the sweepback angle of the lifting-body of hypersonic aircraft should be more than the drag-minimized value. He also proposed a modification to the geometry of the leading edge.
In a theoretical study published in the journal, “Aerospace Science & Technology” in Nov’2005, Prof. Mahulikar proposed a configuration design modification to the hypersonic aircraft’s lifting-body (integrated wings and fuselage). He demonstrated based on mathematical derivations as well as through numerical simulations that due to aerothermal considerations, the sweepback angle of the lifting-body of hypersonic aircraft should be more than the drag-minimized value. For RHV cruising at a Mach number of 7 at an altitude of 35-km, this sweepback angle was calculated to be 79°-80°, i.e. higher than the drag-minimized value of about 73°. Further, the incremental aerodynamic drag for this newly proposed sweepback angle was found to be insignificant.
The geometry of hypersonic aircrafts dramatically differs from commonly seen aircrafts. The lifting-body of RHV that provides the lift (a force that keeps the aircraft in air) is shaped like a surf-board to reduce the wave-drag. In the configuration of RHV shown in Figure 2, the nose-cap has a smaller radius in the side view and a much larger radius in the top view (a non-axisymmetric bi-curvature nose-cap).
Figure 2: Configuration of Lifting-Body of RHV
In studies published in 2017, in Acta Astronautica and Journal of Aerospace Engineering, Prof. Mahulikar and his team computationally validated the earlier proposed theoretical concepts. They studied the aerothermal characteristics of the nose-cap of RHV and Swept-Back Leading Edges (SBLEs) of the lifting-body (front part of lifting-body that first faces the flow). Researchers found that the bi-curvature nose cap heats up lesser than an axisymmetric one (same radii in all directions, i.e. a cap of a sphere).
The numerical simulations were run at a cruise Mach number of 7 and flight altitude of 35-km for different sweepback angles. They indicated that the surface temperature of RHV was high at 1335°C for a design with a sweepback angle of 40° and decreased to 914°C until a sweepback angle of about 79°. Increase in the sweepback angle beyond 79° was found to increase the surface temperatures. The sweepback at which the surface temperatures are the least is the ‘heat-transfer-minimized-sweepback’, which differs in concept and value from the ‘drag-minimized-sweepback’ of about 73°. This ‘drag-minimized sweepback angle’ has so far been the consideration for the aerodynamic configuration design of RHVs.
“The temperatures of the SBLE surface, as well as the upper and lower surfaces of the lifting-body, are significantly lower for the ‘heat-transfer-minimized-sweepback’ than for the ‘drag-minimized sweepback’”, says Prof. Mahulikar. These results have immediate practical implications in the selection of lightweight TPS-materials for RHV. “Therefore, for RHVs, the heat-transfer-minimized sweepback angle of about 80° must be used and not the drag-minimized sweepback angle of about 73°”, he adds.
Researchers also studied how varying the radius of leading edge of RHV’s lifting body can also reduce the temperatures. Conventionally, the leading edge is blunted to reduce the in-flight temperatures. Researchers computationally observed that for 80° sweepback, the leading edge with a smaller radius (i.e. ‘sharper’ leading edge) is at a lower temperature. For sweepback angles greater than about 60°, sharpening the leading edge reduces the temperatures along the SBLE surface. This non-intuitive observation is termed as the “Thermally Benign Sharp SBLE Effect”.
These new findings have important implications for the configuration design of RHVs. But how far are we from actually realizing a hypersonic flight? Quite far, perhaps a few decades, feel the researchers. The next active area of research is the development of an engine that can sustain combustion at supersonic engine air flows and can sustain the high temperatures on its inside and outside. Also, to have a full-fledged operational hypersonic vehicle, it is necessary that bulk manufacturing facilities for its parts, sub-systems, and their assemblage are in place. Until then, we might still have to cramp ourselves for about 18 long hours to fly to the USA from India, hoping to soon fly at hypersonic speeds!
Research papers the article is based on:
1. Theoretical aerothermal concepts for configuration design of hypersonic vehicles
3. Aerothermal Analysis for Configuration Design of Swept Leading Edge Hypersonic Vehicle
4. Aero-thermal analysis of lifting body configurations in hypersonic flow