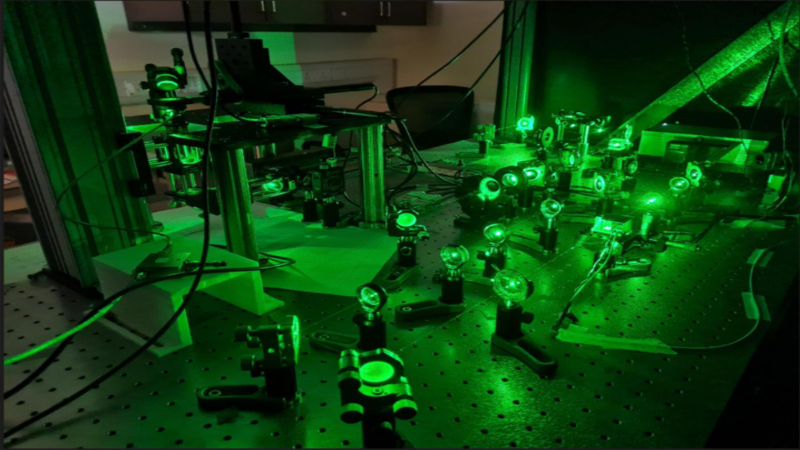
Image: Setup of the experiment (Credit: Prof Kasturi Saha)
Motion picture has evolved phenomenally over decades, giving us a more real-to-life video grab than the choppy and blurry video which we all have grown up watching. This evolution in practice is due to the advancement of imaging technologies over the years, beginning with a modest ~16 frames per second (FPS) video by the 18th century Lumiere brothers to the immersive gaming environments with ~150 FPS. In addition to entertainment, can we utilise this technology to image and understand the human body's complex microscopic workings, such as neuron activity in the human brain?
In an innovative step taken by the Photonics and Quantum Enabled Sensing Technology Lab, Indian Institute of Technology Bombay, the laboratory lead Prof Kasturi Saha and her team exploit the capabilities of a specialised high FPS camera to get time-changing or ‘dynamic’ pictures of very weak magnetic fields, typical of what is found in neurons. A study based on this work was published in the journal Scientific Reports.
We use neuroimaging techniques such as magnetic resonance imaging (MRI), magnetoencephalography (MEG) and functional magnetic resonance imaging (fMRI) for studying the internal structure of the human brain. These techniques measure electromagnetic signals that provide a snapshot of combined neuron activity and help us study specific brain functions. Moreover, these signals are weak, so we need bulky, extremely powerful magnets in these technologies to detect them. Thus, how to increase our ability to detect such weak neuron signals using efficient technology is one of the urgent questions that the neuroscience and medical community at large are striving to answer.
Often, we find imperfections in the crystal lattice structure of diamonds, one of which includes a nitrogen atom located next to a vacancy in the diamond lattice. These nitrogen-vacancy (NV) defect centres have ‘unpaired’ electrons that are extremely sensitive to external triggers like magnetic fields and temperature, making them unique quantum probes to measure weak yet ultra-sensitive magnetic fields at microscopic levels. These changes can be directly measured by sensing low-intensity light, also known as ‘photoluminescence’, emitted from the interaction of these electrons with magnetic fields. The researchers harness these unique properties of NV centres into highly sensitive, atomic-size magnetometers that can map magnetic fields at such smaller physical scales.
The neuronal magnetic fields change much faster than how quickly the current NV-based magnetometers can capture the changes. With the current NV magnetometers, the capture takes a few minutes to a few hours for high-resolution images, and hence anything changing faster than that cannot be videographed and essentially gives us only a snapshot. Prof Saha and her team are the first group of researchers presenting a unique and experimentally efficient improvement by using a specialised readily available ‘lock-in’ camera in a magnetic field microscope setup bringing down the acquisition times from several minutes per frame to an order of 100 FPS. Globally this is the first time anyone has shown sub-second magnetic field microscopy with this technique.
They set up the experiment by mounting a very thin sheet of pure diamond crystal with NV defects on two types of magnetic field sources - a very thin microscopic wire (or a ‘microwire’) and a microscopic coil of wire (or a ‘microcoil’). They introduce variation in the magnetic field by manipulating NV centre spins with microwave frequencies that change on the scale of milliseconds. This rapidly changing magnetic field is caught through the interaction in the NV defect centre within the diamond crystal layer, which results in low-light photoluminescence.
The ingenuity of this experiment, however, lies in how Prof Saha’s team utilised the high FPS lock-in camera to capture the changing magnetic field. As opposed to a conventional camera, in a lock-in camera, each pixel detects only light fluctuations that contain specific frequency or oscillations of a fixed time period and ‘rejects’ light fluctuations of other frequencies. This enhances the signal for the lock-in camera and hence, is suited in ultra-low light imaging scenarios like the photoluminescence from NV centres. As a result, they were able to get a ‘motion picture’ of the magnetic field from the microwire and the microcoil by capturing each change of magnetic field by tuning the lock-in camera to capture the light emitted by NV centres.
Says Madhur Parashar, the lead author of this work, “The experimental imaging setup is a magnetic field microscope, analogous to a standard light microscope. Therefore, it renders the ability to image microscopic (1 micron to around 100 micron scale) magnetic fields found in microcircuits with a current flow and can potentially be extended to imaging dynamic magnetic fields from biological cells like neurons.”
NV-based microscopes thus have the potential to be a promising alternative technology for probing mammalian brain activity. Such a way of pushing the accuracy of sensing electromagnetic fields at the microscopic level using quantum properties of atomic constituents forms the crux of the upcoming new field of ‘Quantum sensing’.
“In the context of India – Quantum Sensing is completely new, and our lab is the first to build such a quantum diamond microscope in the country. We can perform not only biological sensing but also explore quantum materials and many more,” concludes Prof Saha.
This article has been run past the researchers, whose work is covered, to ensure accuracy.