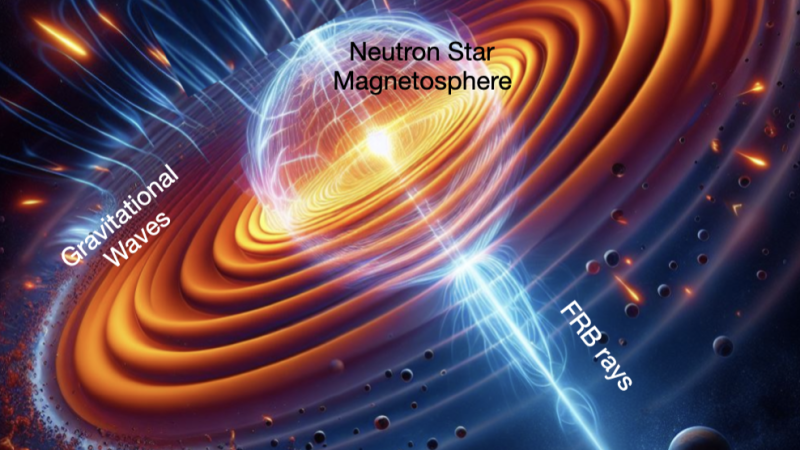
In 2007, American astrophysicist Duncan Lorimer and his students were looking through archival data of pulsars– the pulsing core remnant of a star that has exploded in a supernova (powerful and luminous explosion of a star) at the end of its life. While combing through the data, they noticed a strange spike of energy that lasted just a few milliseconds. They called it a Lorimer Burst, later known as Fast Radio Burst (FRB). It was a short pulse putting out enormous amounts of energy, equivalent to the energy output of our sun in 3 days. Till now, over 700 FRBs have been reported and can be traced to be coming from all around the universe. Some of these, called repeating FRBs, even repeat multiple times, although seemingly randomly and without a discernible period. The origin of these high-energy, transient cosmic phenomena, however, remains shrouded in mystery.
Several attempts have been made to explain FRBs, including some that invoke exotic physics like primordial blackhole mergers and cosmic strings, which are tiny cracks in the fabric of spacetime. However, none of the explanations have comprehensively explained FRBs. For a theory to explain an FRB, it has to justify the three main characteristics of FRBs: its extremely high energy called peak flux, its extremely short timescale of the pulse called pulse width, and the coherent nature of the pulse where the electromagnetic waves are in phase.
At the Indian Institute of Technology Bombay (IIT Bombay), Prof. Shankaranarayanan and his group members, Dr. Ashu Kushwaha and Dr. Sunil Malik (currently a postdoctoral researcher at Potsdam, Germany), have been studying the origin of FRBs. In a new study published in Monthly Notices of the Royal Astronomical Society, they have now proposed a mechanism that explains all three characteristics of FRBs. According to their study, FRBs are generated when high-frequency gravitational waves (HFGW) interact with strong magnetic fields.
“While analysing these bursts characteristics, we found that the natural mechanism of energy conversion from one form to another can potentially explain these observations. When we calculated the conversion of gravitational-wave energy to electromagnetic waves in the presence of a strong magnetic field, all the necessary characteristics were natural outcomes,” remarks Prof. Shankaranarayanan while describing how their model explains the three main characteristics of FRBs.
Gravitational waves are ripples in the fabric of spacetime. Just like a pebble thrown in water causes ripples on the water’s surface, energetic events across the cosmos, like supernovae or the spiralling of cosmic bodies into each other, cause ripples in the fabric of spacetime, generating a gravitational wave. Much like their electromagnetic counterpart, gravitational waves also have a spectrum, ranging from low frequency (kilohertz to millihertz) waves generated by a low energy event like the collision of stars to very high (Megahertz (MHz) to GigaHertz (GHz)) frequencies, caused by extreme events like the collision of small-size black holes. According to the IIT Bombay study, gravitational waves with frequencies in the MHz to GHz range generate short-lived FRBs when interacting with a strong magnetic field perpendicular to the wave.
Their hypothesis pivots on a mechanism called the Gertsenshtein-Zel'dovich effect, where, in the presence of a strong magnetic field, a fraction of the energy of the gravitational wave is converted into electromagnetic waves, like radio waves.
“Gertsenshtein and Zel'dovich identified and studied the energy conversion mechanism in the 1960s and 1970s. It can be understood as ‘energy conversion’ such as converting mechanical energy to electric energy in windmills. Similarly, in the Gertsenshtein-Zel'dovich mechanism, incoming gravitational wave energy converts a fraction of its energy to electromagnetic waves in the presence of a strong transverse magnetic field,” explains Prof. Shankaranarayanan.
The new study from IIT Bombay suggests that neutron stars or magnetars could provide the strong magnetic field required to generate an FRB. Neutron stars are the collapsed core of stars that have 10 to 25 times the mass of the sun and have exploded in a supernova, while magnetars are neutron stars with a very strong magnetic field. It is thought that neutron stars make up nearly 1% of any galaxy, giving us billions of potential sources for an FRB per galaxy. However, the chances of detecting such an FRB event aren't high. The low probability of detection is due to two factors that need to be ensured for it: one, the gravitational wave has to pass through the neutron star or magnetar perpendicular to its magnetic field, and two, the resulting electromagnetic burst has to travel along the line of sight of the observer. In other words, it has to be pointing our way for us to see it.
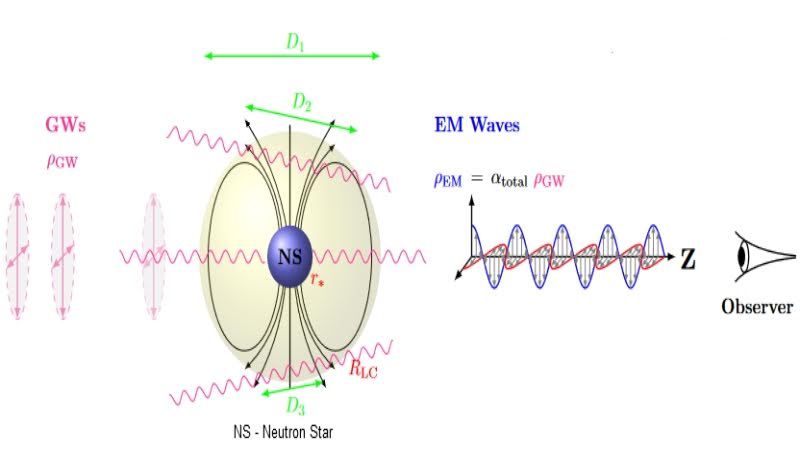
Credit: Prof S. Shankaranarayanan
Their suggested hypothesis could explain a long-standing question in astronomy about the origin of the FRBs and does so without resorting to any exotic physics that invokes theoretical or unobserved phenomenon or objects, like cosmic strings and primordial black holes. Moreover, the mechanism also presents a new way to observe gravitational waves beyond current detection capabilities.
With experiments like the Laser Interferometer Gravitational-Wave Observatory-Virgo interferometers and nanoGrav experiments, humanity has just started probing the universe in gravitational waves, but these are just detecting low-frequency waves with kilohertz (kHz) and nanohertz (nHz) frequencies. Although systems to detect MHz and GHz gravitational waves have been built, like the Australian High-Frequency GW detector or the Japanese 100MHz GW detector, these might take years or even decades to detect an HFGW. The new model presents FRBs as a smoking gun for indirect detection of an HFGW, where observing an FRB would indicate the presence of an HFGW instead of directly detecting one.
The team behind the study has moved onto a more challenging task - explaining repeating FRBs.
“Given that repeating FRBs do not have any particular period for repetition, it is very tedious to explain them. We are thinking of possible ways to extend our model to investigate the repeating FRB characteristics in the future,” conclude the authors.