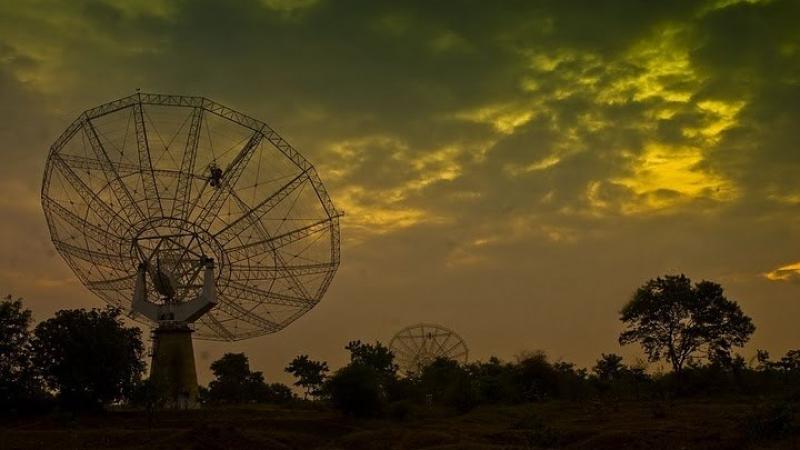
A consortium of astronomers from across the world, including from India, have detected the signature for the background hum of the universe. Called the gravitational wave background (GWB), these are ripples in the fabric of spacetime pervading all of the space around us. The Global collaboration of radio astronomers called the International Pulsar Timing Array (IPTA) made the announcement of the detection on June 29, 2023.
Gravitational waves were first predicted by Albert Einstein’s theory of general relativity in 1916, according to which all of space and time, considered a single entity called spacetime, behaves like a fabric, able to stretch, distort, and even ripple like water. These ripples are produced when extreme energy events take place. Like when massive objects of the mass of stars are accelerating or when stars explode in a supernova. The enormous amount of energy involved in such an event disrupts the very fabric of spacetime, causing ripples that propagate outward in the form of waves. These ripples in spacetime are called gravitational waves. In September 2015, astronomers observed the first direct evidence for a gravitational wave, which was generated when two stellar-mass blackholes (blackholes tens of times heavier than our sun) merged, using the Laser Interferometer Gravitational-Wave Observatory (LIGO).
The gravitational waves, much like their electromagnetic counterparts, have a spectrum ranging from very high frequencies to very low frequencies.
"Imagine two massive objects, like black holes or neutron stars, that orbit each other and eventually merge. When they are orbiting far apart, they start emitting low-frequency, long wavelength gravitational waves. As they get closer, the frequency of the waves also gets higher, while the wavelength gets smaller." says Prof. Manjari Bagchi, a professor at the Institute of Mathematical Sciences, Chennai, and part of the Indian Pulsar Timing Array (InPTA). LIGO and other gravitational wave detectors based on Earth can only detect the highest frequency waves.
To detect the really low-frequency waves, we need much larger detectors—the size of galaxies.
Many low-frequency gravitational waves are being generated by different sources around the universe. Mostly theorized to be a result of the merger of supermassive blackholes (blackholes millions or even a billion times heavier than our sun) like the one at the center of our Milky Way. In fact, our galaxy is currently on a collision course with its neighbor, the Andromeda galaxy. The supermassive blackholes at the centers of both galaxies are stuck in this dance of merger, eventually merging 4.5 billion years later, generating low-frequency gravitational waves in the process.
Much like how raindrops falling in a puddle create many tiny waves that interfere to create a chaotic looking puddle, this stream of gravitational waves from sources around the universe would then interfere with each other and create a continuous noise of gravitational waves that can be detected all around us. This background noise of low-frequency gravitational waves, called the gravitational wave background (GWB), is what the astronomers have now seen signatures of. Its detection, however, required us to turn the entire Milky Way galaxy into a giant gravitational wave detector.
The Pulsar Timing Array and the Galactic detectors
Neutron stars are the remnants of stars somewhat more massive than the sun, once they have exploded in a supernova. The collapsed core of the star that existed is made of densely packed neutrons, packing almost all of the mass of the star into a sphere with a radius of just tens of kilometers. Some neutron stars shoot colossal beams of electromagnetic radiation from their magnetic poles, sometimes stretching light years long. Similar to how a lighthouse is seen when its light is pointed at us,when these beams from neutron stars are pointed in the earth’s direction, we can detect them as a blinking star, called a pulsar.
The pulses of a Pulsar are known to be extremely precise in their period, making them useful as accurate cosmic clocks. This precision of the pulsars is exactly what helped the astronomers detect the GWB.
"The time between the pulses from pulsars is constant. If the pulses are reaching us at different time intervals, then there must be some change in the distance between the earth and the pulsar. Once we model for all other changes in the distance, like the movement of the earth, our solar system, the pulsars, changes to the speed of the pulse while traversing the interstellar medium or while moving close to massive objects, the time interval should remain constant. If there are still any slight changes in the timing between pulses, it has to be because of the change in the curvature of spacetime, which is a gravitational wave," explains Prof. Bagchi.
To make sure it's not an anomaly causing the change in timing, astronomers observe an array of pulsars - 87 to be exact. Hence the name Pulsar Timing Array.
"If there was a change in just one of the pulsar’s timings, then it can be attributed to a change in the pulsar itself, like a starquake (similar to an earthquake but happening on a star) or something. But when all the pulsars are showing a similar change in their timing, it has to be a change in the curvature of space itself, and hence has to be a gravitational wave," she adds.
The low-frequency gravitational waves also have extremely long wavelengths. Which means the distance between the crest and the trough of a single wave can be light years long. To observe the effect of such a long wave on a pulsar, the detectors themselves need to be placed lightyears apart. The solution was to use pulsars, which are far apart from each other and the earth, thus making a galactic sized detector. The team gathered data over a long period of time, in some places for over 15 years, to observe the changes in their timing.
“We need to observe pulsars for long duration as the more data we have, the better we can model out the other effects changing the distances (between us and the pulsar)” remarks Prof. Bagchi
To observe the pulsars spread across the skies, radio telescopes had to be placed around the earth. To achieve this, the International Pulsar Timing Array (IPTA), a multi-institutional, multi-telescope collaboration, was set up. It comprises the European Pulsar Timing Array (EPTA), the North American Nanohertz Observatory for Gravitational Waves (NANOGrav), the Parkes Pulsar Timing Array (PPTA) in Australia, and the Indian Pulsar Timing Array (InPTA). The InPTA is a collaboration between Indian and Japanese astronomers and makes use of the upgraded Giant Meter Wave (uGMRT) radio telescope based in Pune to observe the pulsars.
"It is called the Indian PTA because the uGMRT is situated in India, but the work is being done by scientists from India and Japan. This is truly an Indo-Japanese collaborative effort," exclaims Prof. Bagchi.
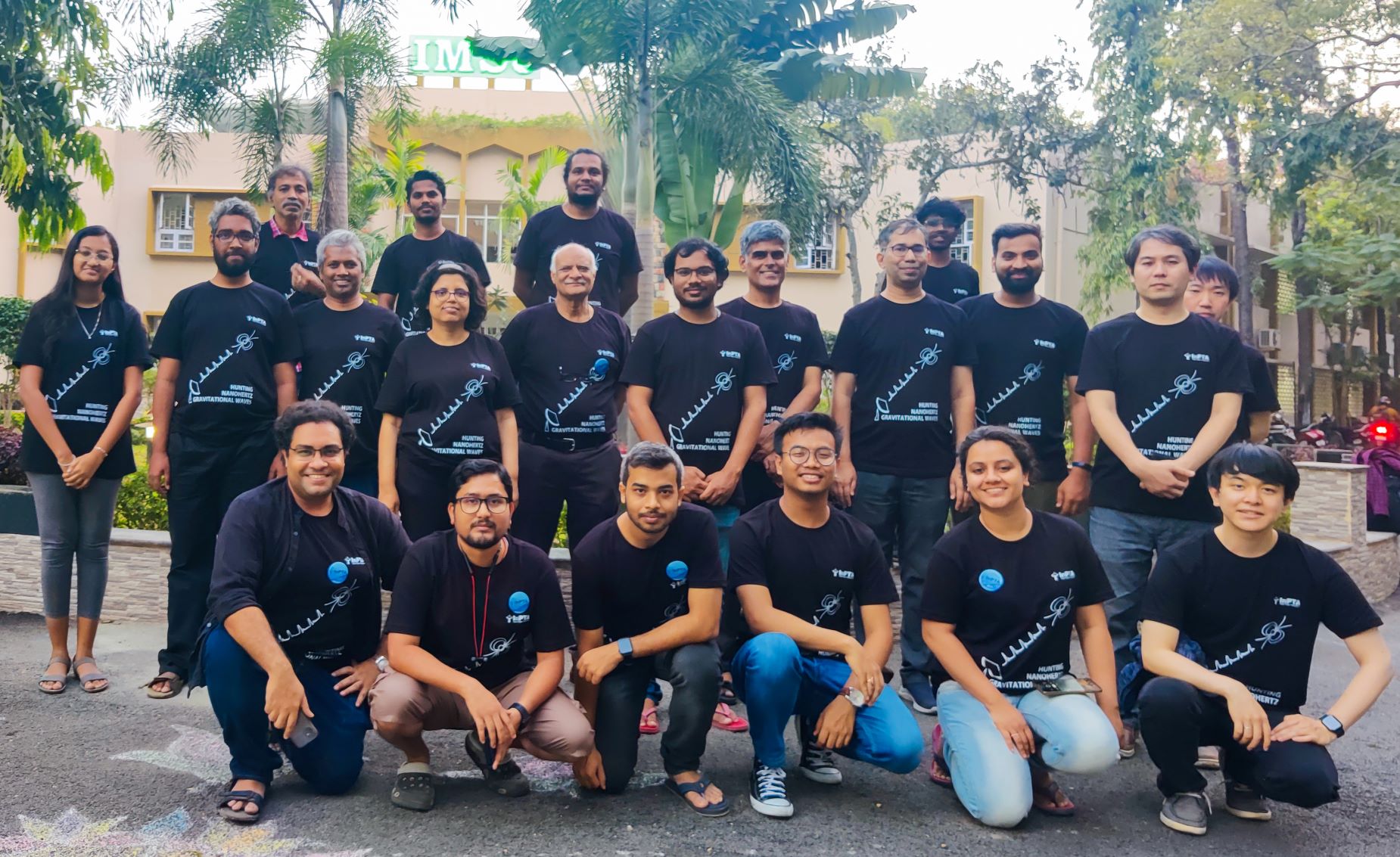
In total 87 pulsars were observed, with NANOGrav observing 68, 32 by the PPTA, of which 18 were common with NANOGrav, and the EPTA and InPTA together observed 25 pulsars, of which 19 were common with NANOGrav and 1 was common with PPTA. Data from the InPTA were combined with those of the EPTA to observe the signature for the GWB. The result agrees with the results of NANOGrav data and the PPTA data.
Looking to the future and listening to the past
The detection of the GWB opens up a whole new set of tools to observe the universe around us. Although we have been able to detect them, our instruments are not yet sensitive enough to gather information about the source of each individual wave from the noise of the GWB. New additions to the IPTA, like the Chinese and African pulsar timing arrays are in the pipeline, which can increase the sensitivity of our detection.
"We will soon combine all our data, including those from EPTA, NANOGrav, and PPTA, which will give us a better understanding of the GWB. Apart from just detecting them, we will also try to understand their sources better." says Prof. Bagchi.
Although supermassive black holes are the leading candidates for low-frequency gravitational waves, they are not the only possible source. Another source could be small fractures in the fabric of spacetime called cosmic strings. These are cracks that were formed during the expansion of the early universe, just a few seconds or minutes after the big bang. The discovery of a cosmic string could teach us about the birth and evolution of our universe, and the GWB could hold the key to unlocking these secrets.
Moreover, such a detection wouldn’t have been possible or would have taken much longer without the global collaboration and data sharing that the IPTA enabled.
"The future lies in combining global efforts. The InPTA too needed collaboration between Indian and Japanese scientists. Even within India, although the uGMRT is being operated by NCRA, Pune, the scientists working on it come from institutes all over the country. So, humanity should work together," concludes Prof. Bagchi talking about the importance of collaborations and open data sharing.
Editor's Note: An edited version of the article was published in Deccan Herald earlier.