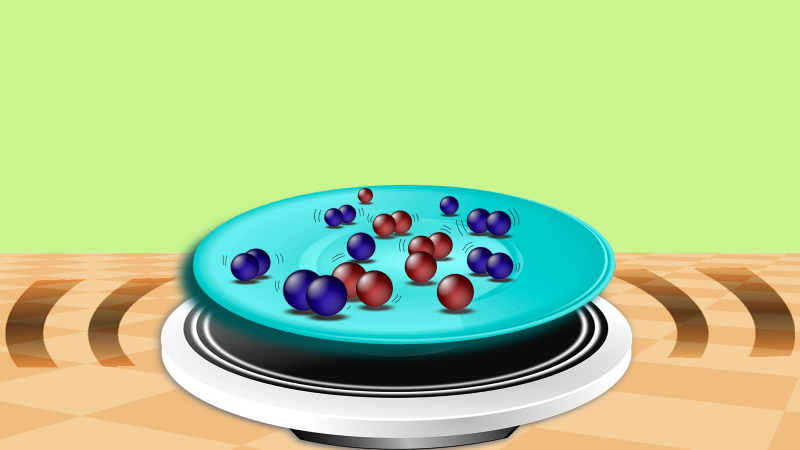
An object can rotate in two ways. For example, a clock’s hands move in what we call the ‘clockwise’ direction. The sense of direction is called ‘chirality’, which can be either left-handed or right-handed, akin to clockwise and counterclockwise. Biological objects, for example, the double-helix of DNA, inherently twist in a preferred direction. Loxodes is a microorganism that swims in freshwater along a spiral, whose chirality depends on the amount of oxygen in the water. The circular trajectories of the bacterium E. coli swimming near solid boundaries or the helical path of sperm cells navigating its neighbourhoods also exhibit chirality.
How the chirality of biological particles affects their motion is not well understood. A recent study by researchers from the Jawaharlal Nehru Centre for Advanced Scientific Research (JNCASR), Bengaluru, and Indian Institute of Science (IISc), Bengaluru, have shown that indeed, chirality of particles makes them undergo special kinds of collective motion, and similarly chiral particles group together. Funded by the Department of Science and Technology, Government of India, their study was published in the journal Science Advances.
“It is challenging to precisely regulate the chirality of biological systems,” explains Pragya Arora from JNCASR, the lead author of the study.
Hence, the researchers 3D-printed oval-shaped millimetre-sized plastic particles, with one side of the beads rougher than the other, creating an asymmetry between its poles. They also designed the beads to have a hollow region on one side of its polar axis and bore a small circular hole on the other side, increasing the asymmetry between the two sides. Thus, they could control the chirality of the particles, and study how it drives their motion.
“3D-printing is an enormously sophisticated technology that gives us direct control of the chirality,” says Ajay K. Sood, professor at IISc and an author of the study.
The researchers classified the 3D-printed particles into six categories depending on the total asymmetry in their structure, which can determine how they behave collectively. Then, they placed beads of each category separately on a plate. “The beads together look like basmati rice on the plate,” says Rajesh Ganapathy, an associate professor at JNCASR, also an author of the study. They then placed the plate under a speaker which they then turned on. The speaker’s vibrations induced vertical motions on the plate, making the beads move and rotate from their original positions and orientations. The researchers recorded this evolution with a camera.
The beads, the researchers observed, formed two kinds of pairs during their motion. When two beads of the same category rotating in opposite directions came together, their individual rotations ceased completely, and they moved together in a specific direction, like one particle. “It is much like two cars in a race with two opposite rotations colliding and then moving forward together,” shares Rajesh. They called these pairs the ‘movers’. When two particles with exactly similar rotations came in close contact, moving in somewhat opposite directions, the result was a pair of particles that underwent rotation only. Hence, they named these pairs the ‘spinners’. The researchers noticed that the movers outnumbered the spinners, and in fact, the categories of beads with a lower amount of asymmetry did not form spinners. By tracing the motion of individual beads that form pairs, the researchers understood why –– the coincidence leading to spinners was much less likely to occur than the one leading to movers.
The researchers then mixed the different categories and observed that the particles selectively pair with other members of the same category, a property they call ‘self-recognition’. Chiral objects in nature often self-recognise resulting in, for example, formation of the double-helix of the DNA and interactions between proteins and DNA. The researchers observed that when the beads of different categories come close, they glide past each other, thus explaining the selectivity. Pairings between different categories, when they do rarely occur, are short-lived, as the individual particles end up separating quickly. Thus, the researchers showed that self-recognition occurs purely due to the particles’ motion, and not any intrinsic attraction between particles.
“We have demonstrated for the first time that objects can self-recognise even when their shape is not chiral,” says Rajesh.
Then, the researchers studied the beads of a particular category, and varied the total density of beads and the total chirality of the group. When the number of left-handed and right-handed beads is equal, the total chirality of the batch was zero. When they were all either left-handed or right-handed, the total chirality was a hundred per cent.
“To achieve this state, we had to flip thousands of beads manually, and sometimes it would take up a full day to set up the experiment,” shares Pragya.
Having recorded the evolution of all the categories of beads, the researchers analysed the data via numerical calculations on the computer. First, they calculated how long the particles took to escape from their neighbouring particles. They concluded that the particles remain caged within their own boundaries much longer if the net chirality is more. The conclusion is independent of the density of beads, which implies that the beads’ motion is directly dependent on the chirality of the group. “I don’t have an answer why,” admits Rajesh.
Probing further, the researchers figured that the particles are also geographically clustered. Moreover, the clustered regions are larger if the total chirality of the group is more. A large cluster indicates that more particles have to move together, thus increasing the time particles take to escape their neighbourhood. “Although this link provides a plausible explanation, we currently lack a clear understanding of why the collective motion depends on the total chirality,” says Pragya. “So, it is now up to the theoretical physicists to explain what we have observed,” she adds.
Chirality is a fundamental property of nature, and understanding how it affects motion in a large number of particles may have larger implications, for example, in complex biological processes. “We plan to 3D-print a small polymer chain and study its dynamics,” shares Rajesh. The researchers want to make even more complex objects, like DNA strands, and study how their structural chirality drives the dynamics of other particles. “The possibilities have really opened up,” Ajay signs off.