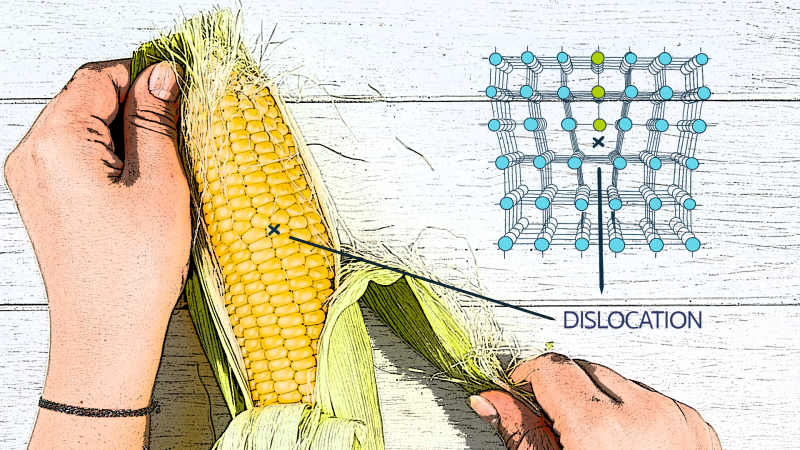
Two new studies from the Indian Institute of Bombay (IIT Bombay), Mumbai, show the importance of defects in the arrangement of atoms in a crystal, called dislocations, in shaping the physical properties of metallic alloys.
Crystals are often imagined as perfect arrays of atoms, or molecules arranged in rows and columns. Yet, perfect crystals are rarely seen in reality. Most crystal lattices have defects, and one of the types of defects is called dislocation. A dislocation occurs when there is an irregularity or a break in the periodic arrangement of atoms or molecules in a crystal. Essentially, it is a sheet of missing atoms in the regular crystal pattern, causing the planes of the neighbouring atoms to shift in order to fill up the space left by the missing atoms.
Although a defect, the presence of dislocations alters the physical properties of the material – a fact that material scientists have been exploiting to precisely engineer the physical properties of a material, such as its strength, ductility and electrical conductivity.
In alloys of iron, such as the molybdenum containing maraging steel, molybdenum atoms are distributed throughout a matrix of iron atoms. When dislocations are present, they act like pipes through which the solute molybdenum atoms can travel much faster as compared to a dislocation free material, in a process called pipe diffusion. The faster travel of the solute atoms through the dislocations aids in faster ageing of the alloy. Ageing, also called precipitation hardening, is a method of strengthening a material by heating it over long periods until it reaches a desired strength. During the heating, precipitates of the solute atoms (in this case, Fe2Mo) are formed throughout the solvent material, thus strengthening the alloy. The more the number of dislocations, the faster the solute atoms could diffuse through the material, reducing the amount of time and energy required to achieve the desired level of ageing.
In their first study, the team from IIT Bombay observed that the shape of the precipitates that formed by pipe diffusion had been altered by faster diffusion of the solute. Precipitates are formed by clumps of solute atoms moving through the material. They were no longer their regular spherical shape but were flattened into a plate-like structure. The team used computer models and simulations to show that as the precipitates grew around dislocation forests, their shape was also altered depending on their interaction with the dislocations.
“The change in the morphology of the precipitates causes deterioration in the alloy’s properties, especially its ductility, which is not a good thing. The study gives us clues as to how to control the prior deformation so as to introduce just enough dislocations to gain the benefits of faster ageing while making sure too many flat precipitates are not produced” explains Prof. Nagamani Jaya, who was part of the study.
For their subsequent study, the team wanted to know how a single dislocation interacted with the solutes in the alloy, specifically during phase separation. Phase separation occurs when two phases separate from a single homogenous mixture. For example, when mixing oil and water, the two liquids undergo phase separation to form separate layers of water and oil. The team wanted to study how the presence of a dislocation aided or affected phase separation in metallic alloys. They once again built a computer model to represent a dislocation within a metallic alloy and ran the simulations.
Phase separation usually happens in two ways – nucleation and growth and spinodal decomposition. Nucleation occurs when a small amount of solute atoms of the alloy accumulates at one point within the mixture. Once this accumulating mass reaches a critical size, it starts to grow. For example, considering Fe2Mo again, molybdenum atoms will accumulate and grow from a nucleation point within the iron matrix, eventually separating the two. Dislocation networks usually provide a favourable site for the material to start the accumulation and thus start the nucleation and growth process. Spinodal decomposition, on the other hand, happens spontaneously where, at particular compositions, the two components of the alloy phase separate, akin to water and oil separating spontaneously into two distinct layers. Although both are well-known phenomena, it was thought that the two processes never occurred simultaneously in the same material.
In their study, the team from IIT Bombay found that both nucleation and growth and spinodal decomposition could happen at the same time within the material. While a single dislocation aided spinodal decomposition, when there were two intersecting dislocations, it also helped nucleation and growth. “We observed that beyond a certain level of composition (percentages of the two metals in the alloy), spinodal decomposition can happen along the dislocation line. But when we consider a dislocation network instead of a single dislocation, at the junction where two dislocations intersect, nucleation also happens. This is the first time in literature, to the best of our knowledge, that both spinodal and nucleation are shown to occur at the same time,” says Arjun Varma R., an author of the study. The team used non-dimensionalized parameters (variables or parameters that are independent of any particular material) for their simulations, which meant the same model could be used to study different metallic alloys.
“When we first saw spinodal and nucleation happening simultaneously, I thought there must be something wrong with our model since we are not trained to think about alloys like this. It took me some time to accept that this was, in fact, true,” exclaims Prof. M.P Gururajan, who was part of both studies.
Their models were further validated by comparing atom probe results (a type of microscopy for studying atomic structures) from literature in Iron-Manganese alloy, which showed evidence of spinodal happening at the dislocations , In addition to this, they have also predicted, using this model, the range of compositions at which there is a possibility of spinodal decomposition along dislocations, for different alloys.
Both studies show us how dislocations play an important role in determining the physical properties of alloys. It allows us to precisely engineer the dislocations to benefit from it while taming its drawbacks. Apart from applications, the studies also give us an insight into the fundamental science of the behaviour of dislocations and their interactions with the atoms of metallic alloys.
Moreover, apart from the lessons in metallurgy, the studies also encouraged the in-house development of code and computer models, which can be used to study other metallic systems and alloys. “Phase field modelling is one of the benchmark problems for high-speed computing. It also takes a long time to run, depending on the type of supercomputer used. Having built the models and written the code ourselves, our group now has the expertise to further improve the understanding of these materials. These skills developed during these studies are one of the most important aspects for us,” remarks Prof. Gururajan.
This article has been run past the researchers, whose work is covered, to ensure accuracy.