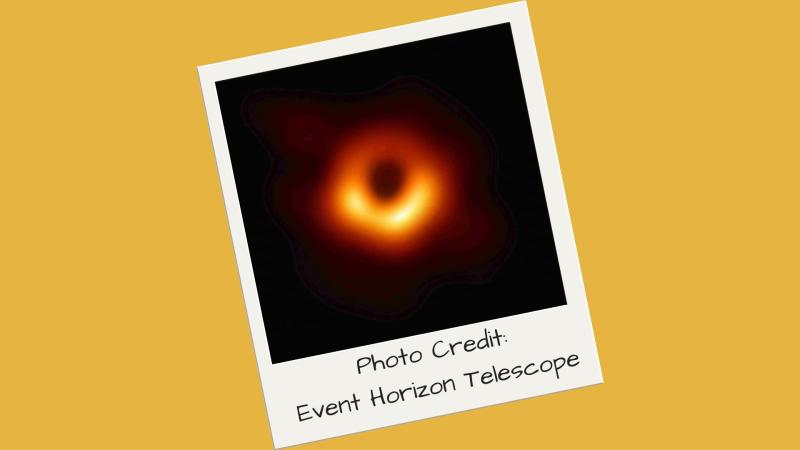
Last month, amidst much excitement, scientists showed the world the first-ever photographic image of a black hole. It looked like a doughnut with a central dark region surrounded by a ring of light. The dark area at the centre of the image, they said, is a supermassive black hole at the centre of the of a distant galaxy called M87 (M stands for the Messier Catalogue), which is about 53 million light-years away. The black hole is estimated to be about 6.5 billion times as heavy as the Sun. The image was produced using the Event Horizon Telescope (EHT), a global network of radio telescopes.
The EHT is an international collaboration of 13 institutions, consisting of several radio telescopes and radio observatories across the world. Radio waves are electromagnetic waves just like visible light but are of much longer wavelength, higher than a millimetre. In contrast, the wavelengths of visible light range from 380 nm to 740 nm. These instruments operate around the clock looking for radio waves from space. These telescopes were built and have served for many years doing astronomy at millimetre wavelengths, studying interstellar molecules and dust, star formation and planet formation. The black hole observations were a repurposing of the existing facilities and lasted only for a few days.
Radio astronomy, a branch of astronomy that deals with studying radio waves emanating from celestial objects, lies at the heart of this incredible feat of imaging a black hole. In a conversation with Deccan Herald, Prof Rajaram Nityananda, a well-known Indian physicist who is now a Professor at the Azim Premji University, Bengaluru, sheds some light on how scientists captured the image of a black hole and the role of radio astronomy in this achievement. In the past, he worked at the Raman Research Institute in Bengaluru (RRI) for over two decades, and later served as the Director of the National Centre for Radio Astrophysics (NCRA), Pune, for nearly a decade.
Comprehending a black hole
Physics tell us that rockets, launched into space, need to travel faster than 11 km/s to escape the gravity of the Earth. Anything that moves slower than this cannot escape the Earth’s orbit, and could eventually come crashing down. However, there are some regions in space where gravity is so strong that nothing, including light, which travels at 300,000 km/s, can escape. Such objects were named “black holes”. John Wheeler if often credited for coming up with the term, but its origin remains uncertain.
John Michell and Laplace first conceptualised black holes in the 18th century, but it was only after Einstein proposed the general theory of relativity that Karl Schwarzschild was able to work out the equations governing their behaviour. Since then, astronomers have found indirect evidence for the existence of black holes from observations of their gravitational interactions with nearby stars and gas. Today, we know that black holes are present at the centres of nearly all galaxies.
An exciting region on the surface of the black hole is the “event horizon”, after which the EHT is named. It is the region of no return—light from just outside the event horizon can escape and reach us, whereas light from inside it, cannot.
If light cannot escape from a black hole, how can we take pictures of them? Supermassive black holes, like the one imaged at the centre of M87, are found at the centres of galaxies. Some of them are continually swallowing gas that is around them. Akin to how an object, thrown from a tall building, gains speed as it falls towards the ground, the gas gains speed, heats up and radiates energy as it falls towards the black hole. This is the “light” we see in the image produced by the EHT.
Seeing the unseen
The excitement behind this first-ever photograph is justified as taking an image of a black hole at the centre of a distant galaxy like M87 poses critical technical problems. Firstly, although the black hole emits many radio waves, very little of it is received at the Earth because of the significant distance between the Earth and M87. These waves are about a billion times less potent than a typical television signal that brings our favourite programme to our living room. Besides, the telescopes we use must be able to distinguish between the radio waves originating from the desired source, the black hole in this case, and other nearby objects. Hence, we need sufficient ‘resolution’ to be able to see objects of the size of the event horizon of the black hole at the centre of M87—about two billionths of a degree on the sky and identify them as distinct objects.
In principle, meeting these requirements would require a telescope much larger than any that can practically be built. In a public talk, organised as a part of the Infosys Prize - Lecture Series, Prof. Nityananda delved into the details of how scientists developed creative solutions to get around these limitations.
“Generally, in radio astronomy, you take signals from many telescopes. Fibre optic cables connect these telescopes. Then you combine the data received by each telescope to help locate and image the source precisely,” explains Prof Nityananda.
The scientists at EHT used a technique known as Very Large Baseline Interferometry (VLBI). The novelty of VLBI is that signals are taken by radio telescopes that are very far from each other, and therefore not physically connected. Instead, the data received by each are recorded and brought to a central processing centre and combined using computers. By combining the data taken by each of them along with information about their geographical separation, the algorithm can emulate a telescope as large as the separation between the telescopes. “It is like having a huge lens, but instead of light coming through the whole lens, only parts of it are transparent. So you get an image with many regions filled in, and you need to interpolate to build the rest of the image”, explains Prof Nityananda.
The EHT consortium used radio telescopes located in Spain, Hawaii, Northern and Southern Americas and the South Pole, to achieve very large baselines, and therefore emulate a telescope the size of the Earth. Making such observations requires exact synchronisation between observatories using atomic clocks.
How does this image change our understanding of black holes? “Hitherto, the models of what is going on near the centre were based on inferences from observations of what is happening further out. Now, if you directly observe what is happening at the centre, the models will also improve, helping us better understand what is actually going on,” says Prof Nityananda, explaining the significance of this photograph. “While many people had expected to see a black hole at the centre of the galaxy, there were also alternative explanations for the observations that have now been laid to rest”, he adds.
Radio Astronomy is gaining traction, and its future is not restricted to imaging black holes. “In India, the GMRT (Giant Metrewave Radio Telescope), based near Pune has been very successful,'' remarks Prof. Nityananda. GMRT is an array of 30 radio telescopes of 45-metre diameter each observing at wavelengths of about one metre. When it was built, it was the largest such array for these wavelengths, “but astronomers are really looking forward to the SKA (Square Kilometer Array)”.
The Square Kilometer Array is an international collaboration, including India, to build the world’s largest radio telescope which will be located in Australia and South Africa. “With SKA, you may be able to see ten times the number of galaxies that we do now. Galaxies form from hydrogen gas, and as you look at more distant galaxies, you are looking further back in time, and you expect to see more hydrogen gas. My colleagues at NCRA and elsewhere have done some of this with GMRT and other telescopes, but SKA will help improve things greatly”, explains Prof. Nityananda. Besides, he asserts that our understanding of cosmological models, dark energy and the origin of magnetic fields will improve with such giant telescopes.
“Finally, SKA will also tell us more about interstellar molecules which might have something to do with the origin of life”, he hopes.