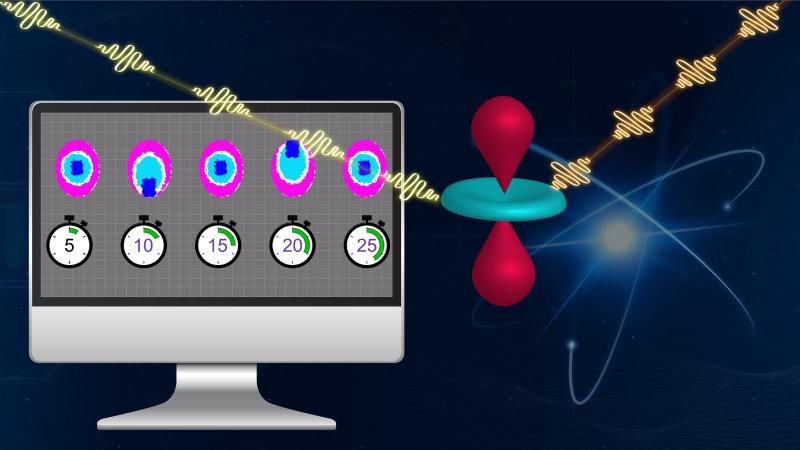
We humans discovered a world of wonders when we were able to see objects smaller than the smallest and events that occurred faster than the fastest. While the universe is said to exist for a billion billion years, what do you think must be happening in the duration when you divide one second in those many parts? A billionth billionth part of a second is called an attosecond, and humans can now generate flashes of light merely a few tens of attoseconds long. Anne L’Huillier, Pierre Agostini and Ferenc Krausz were awarded the Nobel Prize in Physics 2023 for their pioneering work that made attoseconds-long flashes of light possible.
It is exciting to learn that physicist Prof Gopal Dixit of the Indian Institute of Technology Bombay has been actively contributing to the field of attosecond physics.
“I was sure that sooner or later the field of Attosecond Physics would be awarded a Nobel Prize. It is such a fundamental topic in nature as well as has a huge potential to make a big leap in upcoming quantum technologies,” he says.
Prof Dixit and his team are working on several theoretical aspects and applications of attosecond physics. They have been helping solve some of the problems that the Nobel laureates, their teams and other researchers aim to solve.
Attosecond pulses: How to generate them and what they can do
In the 1990s, Anne L’Huillier laid down a path to create short bursts of light that have only a few oscillations and are a few hundred attoseconds long. She bombarded atoms of a noble gas such as neon with a laser beam. When laser light hits an atom, it gives energy to the electrons of the atoms in the noble gas. The electrons absorb the energy and subsequently release it as light with oscillations many times faster than the oscillations of the original light. By carefully designing ways to overlay the oscillations from the atoms, she could create attosecond long pulses. Leveraging the information uncovered by this research, in 2001, Pierre Agostini developed techniques to generate a train of very short pulses, each pulse only 250 attoseconds long. Independently, Ferenc Krausz could generate a single pulse of 650 attoseconds.
The ability to generate a pulse of light so short opened up several possibilities, an important one being able to observe how electrons behave and move in matter. Einstein had predicted that when an atom is hit by light, an electron from that atom is liberated (photoionisation). It was believed that the electron becomes free immediately when it absorbs the energy from the light. In 2010, with the attosecond pulse now possible, Krausz observed and measured the time it takes for an electron to escape from a neon atom. He found that not only is the event not instantaneous—the electron escapes after a few attoseconds—, but also that the time taken depends on the initial energy of the electron. L’Huillier repeated the experiment with argon in 2011-2012.
Matching theory with experimental results
For some time after the experiments were carried out, physicists were not able to reproduce data points in theoretical models and match them with the experimental results. Prof Dixit and team proposed a theoretical method in 2013 that could match two out of three data points in L’Huillier’s experiment, giving confidence to physicists that the experiments are correct and agree with the theory.
Just as an electron absorbs energy from a light pulse and moves to a higher energy state (photoionisation), it can also release energy in the form of light (photoemission) and become attached to an atom (photorecombination). In 2014, Agostini measured photoemission and photorecombination times of electrons in gaseous argon. An underlying assumption he made during measurements was that photoionisation is simply a time-reversed process of photorecombination. Existing theoretical methods could only partially explain the result seen in these experiments. Prof Dixit’s 2015 study could successfully reproduce the experimental data of the photorecombination in argon and established that photoionisation and photorecombination are the same process reversed in time.
“Confirming the validity of Agostini’s experiment gave confidence to the attosecond community that finite time delay in photoionisation and photorecombination are measurable processes,” explains Prof Dixit.
Capturing pictures and videos of atomic processes
When using attosecond pulses to image subatomic phenomena, researchers employ the ‘pump-probe’ technique. The pulse of light used to impart energy to electrons during the attosecond pulse generation process is called the ‘pump’ pulse. Researchers then use another pulse of light called a ‘probe’ pulse to read the pattern formed by the light released by the electrons of the atoms they want to study. They then interpret this pattern to gather information about the events and phenomena related to the experiment.
“Attosecond pump-probe experiments give information about an electron’s motion in time or frequency. However, researchers dream of seeing the entire movie of electronic motion in three dimensions (real-space) and real-time. X-rays can help us look inside solids as they can penetrate surfaces. Time-resolved X-ray diffraction is a versatile approach that helps us observe changes over time. It makes a movie possible, ” comments Prof Dixit.
However, X-rays introduce distortions because they interact with the electrons and make the movie inaccurate. Prof Dixit’s work introduced a method to compensate for this distortion and a more accurate and clean video of the electronic movement could be compiled by processing the images captured.
Prof Dixit also established a method to predict the polarisation state (the orientation of the electric and magnetic oscillations with respect to the direction of propagation) of a generated attosecond pulse. Knowing the polarisation state of the pump pulse is useful for finding specific things about objects being imaged—for example, finding out if a molecule has a left-handed or a right-handed arrangement of atoms inside it. Some molecules (called chiral molecules) can have the atoms forming them arranged in two different ways. The mirror image of one arrangement cannot be superimposed on the other arrangement, just like our left and right hands. It is important to know the exact arrangement because while one can be very useful, such as a medicinal drug, the other could be at best ineffective, and at worst toxic.
In another work, Prof Dixit and team used the pump-probe technique to observe how electric charge is distributed inside a molecule to help visualise the movement of electrons in molecules. The finding can help understand how electrons are exchanged when complex chemical reactions occur.
One of Prof Dixit’s recent studies also explores the electric charge movement in compounds whose molecules have five-membered rings that contain carbon and other elements.
Improving Attosecond pulse sources
The initial methods to generate attosecond pulses used gases and involved bulky and expensive apparatus and complex methods. Putting the attosecond pulses to use required more control over the duration, frequency and polarisation of the pulses. The light sources also needed to be portable and easy to use.
Prof Dixit’s team is working on schemes to generate attosecond pulses using solids.
“Generating attosecond pulses using solids is a game changer for attosecond physics and photonics as the setup would be compact and generate intense attosecond pulses. In future, it could be possible to buy a compact attosecond laser generator from a vendor, just like it is possible to buy other laser sources just now,” hopes Prof Dixit.
At the same time, he has put forth a theory that can enable generating pulses with the intended polarisation of light.
Attosecond-driven quantum technology
Even as computers are getting faster and faster, the speed at which each step in computing occurs—called the clock speed—has reached a limit. Quantum computing is considered as a technology that can overcome speed limitations and offer a multifold increase in computation speed.
A theoretical study from Prof Dixit’s team has established that a property of electrons called spin, which can be ‘up’ or ‘down’, can be potentially used to generate very high-frequency oscillations that can be used as a clock for computers. Just like introducing controlled impurities in silicon made complex and high-speed electronic circuits possible, introducing specific defects in specific materials can alter the spin of the material. When light is shone on such materials, the electrons generate oscillations at frequencies much higher than the original light.
“For the first time, we have shown that the spin of the electrons can be used to control their oscillations at frequencies that are much higher than the current frequencies. This can help in improving the clock speed of processors by at least a thousand times by shining laser pulses on carefully defected materials,” says Prof Dixit.
Another recent research from their group explores an optical method to detect and control another property of electrons, called valley states. Valley states can be used to represent units of computation or qubits in quantum computing. Faster detection and control of qubits can help improve the speed of quantum computing.
“The ultimate aim of any research is to improve the living standard of human life. Attosecond physics can help chemists design new molecules, may make tabletop quantum computing at room temperature possible, and may help detect cancer at an early stage,” concludes Prof Dixit.