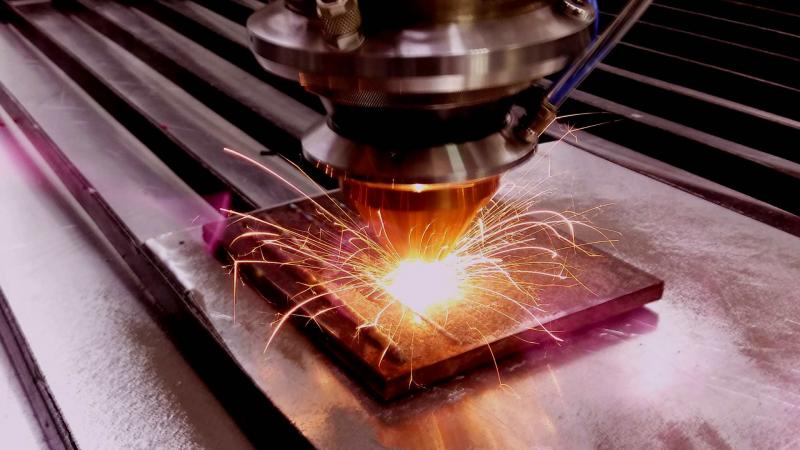
Study proposes an improved additive manufacturing method to restore damaged components
Does it not break your heart when you have to replace an expensive possession just because of some small damage? Many would certainly prefer repair and reuse, if possible! It is no wonder that ‘reuse and recycle’ is the often recited slogan for sustainable consumption of many goods. In the aircraft industry, industrial components, engine parts and even moulds required to make them, undergo wear and tear—mostly on the surface and subsurface—after a period of operation. However, for these crucial and high-cost components, we must also have reliable ways to repair them, to prolong their lives.
Laser additive manufacturing is an upcoming option to restore damaged components in automobiles and aircraft industries. In this process, laser light is focused on the cracked area, which heats and melts a thin layer of the surface to create a melt pool. Powdered metal, is then supplied in the melt pool using a nozzle, which easily bonds with the material of the component after solidification, thereby repairing the damage. Repairs done with this process can be localised, easily automated, and are more precise compared to those done using other methods like welding.
In a study published in the journal Scientific Reports, researchers from the Indian Institute of Technology Bombay and the Australian Nuclear Science and Technology Organization (ANSTO), Sydney, Australia, have developed a computational model to improve the laser additive manufacturing process. The model estimates the optimal height of the newly deposited layer of fused metal such that the restored component does not develop cracks after repair.
“This process is economical, both in terms of consumption of energy and cost, thereby enhancing sustainability. It will help in the sustainable restoration/remanufacturing of expensive and critical aviation parts, turbine blades, diesel engine pistons and moulds”, explains Prof Ramesh Singh, one of the lead researchers of the study.
With the automobile and aircraft repair industry projected to grow by 18% in the next five years, repair and remanufacturing of industrial products can reduce the human impact on the environment as well as increase economic growth.
Any repair process that uses thermal energy to heat the component induces residual stresses in it after cooling. One of them is tensile stress, which tends to pull the material apart, and the other is compressive stress, which is the opposite and pushes the material together. If not controlled, these stresses can be detrimental to the life of the restored component. While tensile stress can cause cracks on the surface, compressive stress can delay or even prevent the propagation of such cracks. Often, residual compressive stress increases the life of the component.
When stresses induced during the process make the components susceptible to cracking and damage, it defeats the purpose of repairing the faults. Using a trial-and-error approach to get everything right would be too costly and time-consuming. The computational model proposed by researchers of the current study addresses these challenges. Using simulations, it can predict how the laser heating affects the thermal, mechanical and metallurgical properties of the component material.
Since only the damaged part of a component is heated using the laser, there is a large difference between the temperature of that region and the rest of the component. As a result, the deposited layer has a tendency to shrink upon cooling which is resisted by the rest of the component, giving rise to tensile stresses as the material solidifies. The researchers considered metallurgical changes in their model along with thermal interactions to better predict the stress in the deposited layer.
The input parameters for the model include the rate at which the metal powder is fed and the diameters of the nozzle and laser beam. It then calculates an initial value of deposition height, the corresponding speed of laser movement and the stresses in the deposited layer and those between the layer and the substrate. The researchers simulated different deposition scenarios by varying the input parameters. To validate the model, they used it to calculate the residual stresses in components made of steel and restored with high vanadium content crucible steel, and cross-checked the predicted stresses with the actual stress values measured in experiments carried out at IIT Bombay and ANSTO Sydney.
The height of the deposited layer needs to be just right. If it is lower than the optimal value, it can result in tensile residual stresses at the region where it fuses with the previous layer. On the other hand, if it is high, more metal powder than required will mix with the molten base and impact the structural properties of the surface, such as making it brittle.
By using the model, the repair process is continued if the predicted stress is compressive in the deposited layer, and there is no tensile stress between the layer and the substrate for this height and laser power. Otherwise, the input parameters are modified to obtain a new height, and the entire process is repeated until these conditions are met. The researchers plan to facilitate the industrial use of the proposed model to repair components, using robotics.
This article has been run past the researchers, whose work is covered, and the institution to ensure accuracy.