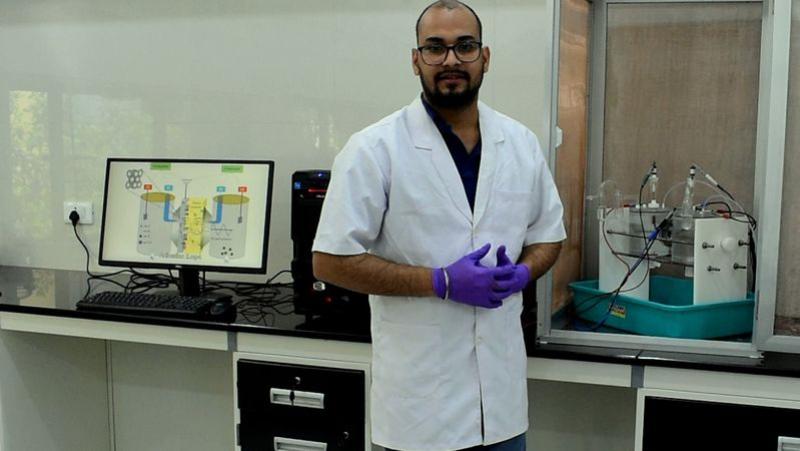
How often have we not encountered corrosion in some of our devices? Although there would be some coating to prevent them, the performance of the coated material deteriorates over time. These can affect the overall performance of the device itself.
A key process responsible for this is the oxygen reduction reaction (ORR), one of the fundamental processes in electrochemistry. This is responsible for reduction of molecular oxygen to water that occurs at the cathode in various electrochemical devices like fuel cells, metal-air batteries, and electrolysers. In other words, the oxygen molecules from the surrounding environment react with electrons and hydrogen ions to form water.
Scientists have been studying the interactions at the interface between the coating and cathode. In practice, there are established methods to evaluate viability of a coating for corrosion protection. However, an improved quantitative method to characterise the coating performance was lacking.
In a breakthrough for electrochemical research, scientists at the Indian Institute of Technology Bombay (IIT Bombay) have combined a recently developed hydrogen potentiometry (HP) method with conventional electrochemical impedance spectroscopy (EIS) to build an innovative approach for measuring the coating performance. Their method allows quantifying buried interfacial reaction rates. This pioneering technique offers a deeper understanding of how reactions occur at the interfaces between materials, which has significant implications for various fields, including energy conversion and corrosion prevention.
“Since the interface is buried, a complementary non-destructive electrochemical technique to monitor in-situ interfacial changes and provide quantitative information to co-relate and deepen the fundamental understanding derived from the hydrogen potentiometry method was needed. My postdoctoral work on using non-linear EIS to characterise the degradation of Li-ion batteries gave me the idea to combine it with the HP technique to build a robust approach to measure ORR kinetics,” says Prof Dandapani as the motivation behind this research.
Mr. Rasmi Ranjan Tripathy, PhD scholar and the first author of the paper, notes that the choice of an alkaline electrolyte is very critical and has a two-fold purpose.
“It allows for stable steady-state hydrogen charging currents on the back side of the hydrogen permeable membrane vital for ensuring dynamic electrochemical equilibrium conditions on the front side. This was tough to achieve using an acidic electrolyte due to adsorbate related challenges. Secondly, for real life applications on industrial metals such as iron and zinc, only an alkaline electrolyte will work as it allows the formation of a thin passive layer and prohibits active metal dissolution typical in an acidic environment that contributes to unwanted side reactions,” explains Mr Rasmi Ranjan Tripathy.
The experiment involved a model interface made of PMMA (poly(methyl methacrylate)) and Pd (palladium) where the inhibited oxygen reduction reaction (ORR) kinetics were observed. The presence of a physisorbed polymer at the buried interface caused a cathodic shift of 50 mV in potential compared to bare Pd. This shift was clearly visible in the I(U) curve, a graphical representation of current versus potential that can be derived from the hydrogen potentiometry (HP) method. Furthermore, electrochemical impedance spectroscopy (EIS) revealed a 26-fold decrease in the RCT (charge transfer resistance) value, which reflects the inhibiting effect of the physisorbed polymer.
In a subsequent analysis of a severely inhibited interface, the scientists applied octane thiol as a self-assembled monolayer on Pd, with a topcoat of PMMA. This configuration led to an even more pronounced cathodic shift of 190 mV compared to the model PMMA/Pd interface, indicating a significantly inhibited reaction. The corresponding EIS measurement showed a 100-fold decrease in the RCT value, confirming the heightened inhibition.
The ground-breaking aspect of this combined approach lies in its interface sensitivity, which enables scientists to anchor specific functional groups to metal substrates through chemisorption. This opens avenues for quantitatively measuring ORR rates and assessing the effectiveness of protective coatings in real-world applications. For instance, thin layers of industrially relevant metals, such as iron and zinc, are currently being deposited on the exit side of the Pd membrane over which polymer coating will be bonded to mimic an interface that is of commercial interest. This will allow the technique to be used as a standalone tool for providing crucial input to simulation programs, aiding in the selection of appropriate coatings for various practical applications.
“The primary aim of these kinetic measurements is to provide input data to a computer program that can predict the corrosion rate of organic coatings. Such simulated coating lifetime will guide in appropriate choice of polymer coating for on-field application in the mobility, oil and gas pipeline, infrastructure, marine and packaging industries. More importantly, we believe this approach could go beyond corrosion related applications and find use for example in hydrogen sensing where the ability to quantitatively measure atomic hydrogen induced interfacial electrochemical changes can be exploited. With the growing interest in transitioning to a hydrogen based energy economy, this work finds relevance and scope for future research in applications related to hydrogen in materials,” says Prof Dandapani emphasising the importance of this work.
These advancements in understanding interfacial reactions provide researchers with invaluable insights into the behaviour of materials and their performance in electrochemical systems. With further refinement and application, this novel combined approach has the potential to revolutionise fields such as energy conversion, corrosion prevention, and material science, contributing to more efficient and sustainable technologies.
As scientists continue to explore the hidden intricacies of interfacial reactions, we move closer to unlocking the full potential of electrochemical processes and paving the way for innovative solutions in diverse areas of science and industry.