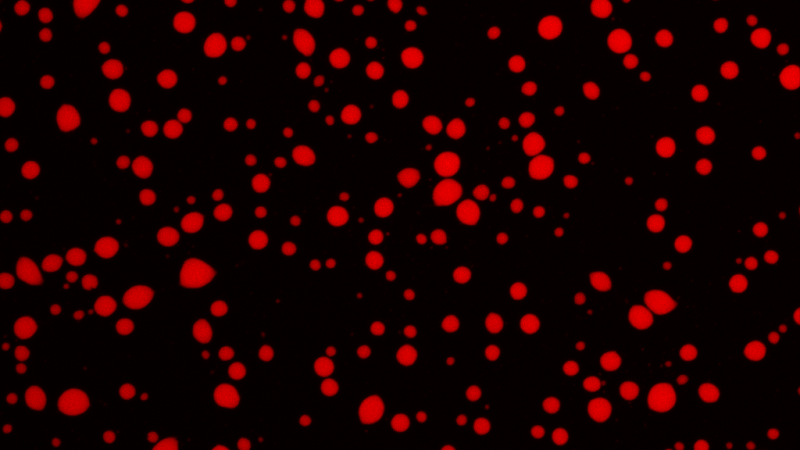
Liquid-liquid phase separation (LLPS) occurs when a homogeneous solution of (bio)polymers phase separates into distinct, coexisting liquid phases under certain conditions, like the phase separation of oil in water. In living cells, LLPS plays an important role in the formation of bodies called membraneless organelles, like nucleoli, Cajal bodies (distinctive structures found in the nucleus of a cell), nuclear speckles, and stress granules, where proteins and other biomolecules undergo LLPS.
In cells, protein LLPS can be beneficial under normal physiological conditions, but there are instances where it can lead to malfunctions and diseases.
“Protein phase separation has garnered significant attention in the context of neurodegenerative diseases such as Amyotrophic lateral sclerosis (ALS), Alzheimer's, and Parkinson's, where LLPS has shown to be the nucleation mechanism for toxic protein aggregation. Therefore, LLPS contributes to the understanding of the mechanism of disease and developing treatments against them,” remarks Prof. Samir Maji, a professor at the Biosciences and Bioengineering department at Indian Institute of Technology Bombay (IIT Bombay).
Although we understand its importance, scientists could not confirm as to what caused proteins and other polypeptides (building blocks of proteins made of amino acids) to undergo LLPS.
Conventional theory regarding ‘phase separation of proteins and polypeptides’ says that the ability to phase separate is inherent in their amino acid sequence and structure. Now, new research from Prof. Maji and his team at IIT Bombay has questioned this long standing hypothesis. Instead, it suggests that all proteins and polypeptides, regardless of their sequence and structure, can undergo LLPS, but only under distinct circumstances.
The theory indicates that at a certain threshold concentration of macromolecules, like proteins and polypeptides, the interactions between the different macromolecules increase. This activity leads to more complex interactions that eventually cause the proteins and other macromolecules to condense out of the solution, referred to as biomolecular condensate formation.
“We proposed that condensate formation is an intrinsic property of proteins/polypeptides irrespective of their sequence or structure; rather specific conditions such as increased protein concentration increase the extent of intermolecular interactions, which is sufficient for inducing LLPS,” explains Prof. Maji.
The environment inside a living cell is a crowded place, filled with proteins, nucleic acids and other biomolecules. This phenomenon is commonly known as molecular crowding. Researchers use a class of compounds called molecular crowders, which can replicate this crowding phenomenon.
According to Prof. Maji “a macromolecular crowder such as polyethylene glycol, ficoll, or dextran, mimics the intracellular crowding and increases the local concentration of macromolecules influencing the formation of condensates”.
Using the macromolecular crowder polyethylene glycol (PEG-8000) to increase the concentration of the macromolecules in the solution, the team examined 23 proteins/polypeptides with various structures and sequences. The selected protein was mixed in with the crowder in a solution and observed for the formation of liquid condensates, which signifies phase separation taking place. Their findings revealed that all proteins and even some highly charged polypeptides have the potential to form liquid condensates. But these formations have very different phase regimes (concentrations requirement), and are dependent on various intermolecular interactions.
Three forces, namely electrostatic, hydrophobic, and hydrogen bonding, were found to be playing an important role in inducing intermolecular interactions. Electrostatic is the force between any two oppositely charged particles. Hydrophobicity is the ability of molecules to repel water molecules and hydrogen bonding is a weak interaction between molecules resulting from the attraction between hydrogen atoms and other electronegative/negatively charged atoms in molecules. The team showed that any or a combination of these intermolecular interactions play fundamental roles in individual protein/peptide LLPS.
Presence of electrostatic forces between charged segments of a macromolecule leads to increased interactions between the macromolecules, which promotes phase separation.
“In addition to electrostatic interactions, the exposed hydrophobic surface is highly susceptible to intermolecular interactions and can drive protein LLPS. Apart from these, intermolecular H-bonds can also be attributed to interactions governing phase separation,” adds Prof. Maji.
The interplay of these various intermolecular interactions govern phase separation, confirming that LLPS is indeed a generic phenomenon of proteins/polypeptides in a particular environment.
Although we have just seen a glimpse of the complexity of protein LLPS, the team behind the study are already planning their next steps to explore further.
“Our next step is to look for a common principle governing phase separation of protein pairs in a multicomponent system,” says Prof. Maji about the future of his research on protein LLPS.
The new research could provide key insights into this important biological process. It could aid in the development of new and effective ways to identify disease causing malfunctions in LLPS and stop them before they progress.
“The potential to prevent the disease progression at the early stages offers hope for effective treatments in the future,” remarks Prof. Maji.
More importantly, the research provides a new dimension about an important biological process of phase separation. Future studies of the phenomenon can significantly bolster our understanding of cellular machinery and protein behaviour.