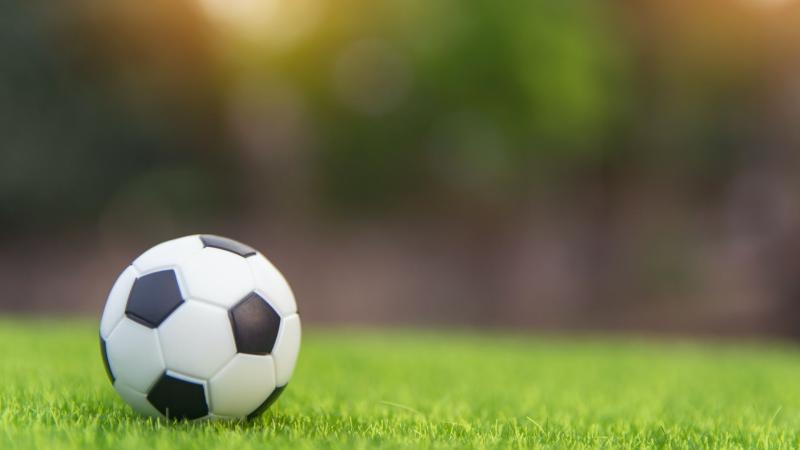
[Image by Tevarak Phanduang on Unsplash]
Have you ever wondered why the surface of a football is stitched together with smaller pentagons and hexagons, even though no floor tiling is ever pentagonal? Mathematicians have long known that tiling floors, which are usually flat, by using pentagons will inevitably leave out distinct gaps. However, the situation is different if the surface is curved, like that of a ball. Solids, like the football here, have a repeated structure of its constituent atoms or molecules.
Liquids, on the other hand, are different. They have randomly-distributed molecules without any periodicity in their positions. On a flat surface, they flow freely, with a varying density in different locations at different periods. Glass is a phase of matter that exhibits rigidity like solids but does not have a periodic structure, resembling liquids. Above a certain temperature, when glass is confined to a flat surface, it transitions into a liquid, and this is called the glass transition. What happens if liquids are confined to a curved surface, like a sphere, instead? Would they show changes in their density like solids? Would glass transition still occur if the glass is confined to a spherical surface?
A new study has now answered these questions through experiments with liquids and glasses confined to spherical surfaces. The study, published in the journal Nature Communications, was conducted by physicists from the Jawaharlal Nehru Centre for Advanced Scientific Research (JNCASR), Bengaluru, and Indian Institute of Science (IISc), Bengaluru. It was funded by the Council for Scientific and Industrial Research (CSIR) and the Department of Science and Technology (DST), Government of India.
“Some of the best minds have grappled with this problem, and we are just beginning to address it through experiments,” says Rajesh Ganapathy, an associate professor at JNCASR and a co-author of the study.
He is also a recipient of the recently-announced Shanti Swarup Bhatnagar Prize, India’s most prestigious award in science.
The researchers designed novel experiments in the laboratory to study the behaviour of liquids and glasses on spherical surfaces.
“There are more theories of glass transition than there are theorists who propose them,” David A. Weitz, a professor at Harvard University, had once joked.
“Different theories predict different outcomes, hence experiments are very important,” shares Rajesh.
As the spherical surface for their experiments, the researchers chose emulsion droplets of oil in water and glycerol. These droplets had diameters of about 30 microns. They then designed colloidal particles, made of micron-sized polymers. When inside the emulsion, these colloidal particles stuck to the inner layers of the emulsion droplets by electrostatic attraction.
“Designing the correct setup took us close to one and a half years,” says Navneet Singh, a PhD scholar at JNCASR, and the lead author of the study.
The researchers tried various combinations of the oil and water mixture, with different amounts of the colloidal particles, to get them to stick to the inner surface of the emulsion and to make them flow.
“We knew certain physical parameters of these various components, which guided our search for the correct combination,” adds Navneet.
Using a microscope that could capture sharp images, the researchers took two-dimensional snapshots of the spherical drops at different heights. They later collated these images to create a three-dimensional time-lapse video that could help them in observing how the colloidal glasses or liquids flowed over the surface of the emulsion. The researchers found that the liquids behave exactly like how theories predict them to, in three-dimensional space. This implies that the curved space of the spherical emulsion, which is two-dimensional, makes the liquids behave as if they are in three-dimensional space. Where is the third dimension coming from?
Theories have predicted that liquids or glasses will show variations in their densities if confined to a flat two-dimensional space.
“We do not see any signs of these fluctuations, which makes the behaviour of the colloidal particles akin to three-dimensions,” says Ajay Sood, a professor of IISc, and a co-author of the study. “The curvature has thus effectively added an extra dimension.”
The researchers hoped to see the transition of the colloids from the glassy phase to the liquid phase, as predicted by several theories, by varying the number of colloids attached to the surface of the emulsion droplet. Whereas physicists have observed this transition on various flat surfaces for different colloids, this is the first time anyone has attempted to see it on a curved surface. The transition from a glassy state to a liquid state happens exactly as the theories predict, the team found.
“The experimental results are extremely reliable and reproducible,” asserts Navneet, who repeated this experiment after returning to JNCASR post the COVID-imposed lockdown.
As a next step, the researchers plan to conduct more such experiments to test out the various theories regarding how liquids behave on spherical and other complex curved surfaces. Devising such surfaces and attaching the colloidal particles onto them can take them multiple trials, and the process has begun.